Editor: Terry Hancock
Deep Space Shipping
Right at the heart of every space project is the issue
of getting us (and our stuff) into space. As we move beyond expeditions,
such as the Apollo program was, we will find a need for reliable shipping
from planet to planet.
This will require a lot of development, but as our
special guest writer this month shows us, there are already paradigms
we can follow for designing a future space freight service.
By Paul Seitz
Mr. Seitz has over six years experience in the operations
of an ocean-going container-shipping business.
Now that human presence has nearly covered every square inch of the
Earth, there are those with vision who would call on humans to join them
in expanding the human community on into space and to other worlds. The
International Space Station is inexorably stepping toward
completion and Mir station has become an icon of private
enterprise in space. Following these first steps many societies around
the world are focusing on building establishments on the Moon and Mars.
Any off-world settlement will be highly dependent on the transportation
of supplies from the Earth. Certainly, for the continued growth of settlements,
bringing commodities and goods to and from the Earth will be a necessity.
A reliable, efficient and cost effective method of transportation will
be utterly necessary for survival. Such a system is currently in place
and operating in one of the harshest environments on earth: the open ocean.
The basic concepts that built this Earth-bound network may be transferred
to a space-bound freight system. In so doing, private enterprise need
not begin from square one, but may learn from, adapt, and improve existing
operations -- thus giving a jump-start to a potential off-world industry.
Just as ocean liners ply deep-water trade routes, a freighter company
in space would connect the longer distances between settlements. Supplies
coming from Earth could be lifted via multiple entry vehicles and warehoused
at an orbiting station. Once enough supplies are accumulated, the space
vessel would receive and transport the items to the lunar base or bases.
Items from the colony destined for Earth would be picked up, transported
to the station, and finally brought back to Earth in the reverse manner.
The Cargo Containers
Taking a page directly from ocean liner operations, supplies would be
most efficiently transported in standardized containers rather than as
individual items. Such containers would at first need to be small, as
the market demand would be low. However, the establishment of dimensions
should keep a firm eye on the future and bear in mind either longitudinal
or width expansion to accommodate the rise in demand and subsequent rise
in cargo volumes.
An example of possible dimensions would be a 1-by-1-meter cube to begin
with. As demand increases, a 3-by-3 meter cube and a 3-by-6-meter box
might be added. Containers may then be filled with any items, reused,
and easily stowed on the vessel. Contrary to the ocean operations case,
stowage weight would not be a factor, but mass and volume would. Each
container may be easily identified by such factors and calculations could
be swiftly administered.
Construction may very well follow the blue prints of what is currently
used on the oceans today: two doors on each end, slightly rippled sides
with reinforced edges, and four corner posts for securing to each other
container and to the vessel. The cargo containers might be built in factories
on Earth in a collapsed state, thus making it easy to transport them through
the atmosphere and fully assemble them on-orbit. An additional step would
be to make these containers able to collapse and assemble on demand. When
the container is not being used, either at station or the moon, the containers
waiting to be used would not take up much additional volume. Such design
decisions would need to take into consideration strength verses cost.
The Vessel
Whereas the creation of a space station requires an atmospheric re-entry
vessel to maintain the supply flow to and from Earth, the establishment
of a lunar colony creates the immediate need for a dedicated space vessel.
There are perhaps two methods of obtaining such a vessel:
The first method would involve working with pioneering companies wishing
to establish themselves permanently or semi-permanently on the moon. These
companies would construct a vessel that would be specialized to their
needs. The schedule of such construction would be in line with the company's
overall startup goal. A transportation company could then offer to lease
the ship from the pioneering company, thereby producing an immediate return
on investment for that pioneering company's capital outlay. The transportation
company, by leasing the vessel, would take on the responsibility of operating
the ship. Of course, any such agreement would undoubtedly contain the
byline that the transportation company must meet the requirements of the
vessel owner in terms of cargo carrying capacity. However, the opportunity
that this opens, is that any excess space could be sold to third parties.
By so doing, the transportation company may avoid construction delay and
cost, while the pioneer company obtains the ready return on the vessel
construction costs.
The second method, would be to construct a vessel from scratch. In space,
there is a unique freedom of design available for optimal commercial usage.
On the ocean, every vessel must be large enough to accommodate all cargo
for a particular service: as demand increases, so must the size of vessels
-- at considerable costs of construction. A company must in fact build
on the expected demand, producing even larger ships than what are currently
needed. Along with this concept is the idea that in the course of a year
market demand may rise and fall due to quotas, seasons, fiscal years,
and any number of other factors, yet the size of vessel must remain the
same. Given that vessels are over-sized for peak season, there is much
unused and wasted area on board the vessel at slack market.
In space, this could cease to be. The creative design of dedicated cargo
carrying vessels, enables a situation in which the vessel is fully utilized
with no wasted space on board, whether at peak season or slack. This is
because the vessel could consist of a bridge, crew accommodations, and
engine, without cargo bays. The cargo, consisting of standardized containers,
may be secured in such a way that it becomes a part of the vessel. In
essence, the size of the vessel would flux every time cargo is received
or discharged. Additionally, by building such a minimalist vessel, construction
cost would be greatly reduced.
All the common methods proposed for space operations: docking on-orbit
with a space-station at Earth, and either doing the same at the moon,
or landing, or using landers (according to the size of the vehicle), have
direct analogues to what is used on the ocean today: Vessels that can
enter ports safely do so and dock alongside the port on their own. Larger
vessels are met as they approach the port and are safely assisted to the
dock by small tugboats. Very large ships find it necessary to anchor just
outside the port and transfer cargo to smaller vessels.
At first, a station-lunar service would depend solely on the transit
time between the two locations. A service with one vessel could make calls
every two-weeks, which initially may be able to meet demand. However,
as population increases, so must the size of the service. The construction
or acquisition of a second vessel would enable once per week calls. A
weekly service would allow just enough time for the local accumulation
and distribution of cargo from the loading/discharging warehouses.
Freight vessel crews
Automated ocean vessels have been investigated in recent times, yet
no move to complete such a vessel has been made. Cost and safety are the
two main factors against such automation. The same would hold true for
dedicated space vessels. The crew of such a vessel provides an invaluable
check on systems. Repairs can be undertaken immediately and unexpected
situations may be reckoned with. This being said the number of crew on
board would be dependent on the design of the vessel. At this point in
time an estimate of three crew would be reasonable.
Along with the relief crew then, total crew would be six. Crews would
stand six-month shifts with six-month relief periods. As earth launch
costs decrease more frequent crew changes may be arranged but this may
be considered an option given by each crew pairs. In other words a particular
crew member may come to agreement with his relief to swap places every
three months instead of every six.
Terminal Operations
The on-orbit collection station, would in effect be a consolidation
warehouse. Shipments from any launch site around the world may be received
at any time.
Shipments may be in breakbulk style or in any form as suitable to multiple
entry vehicles. All the cargo may then be collected and stored until the
next freight ship departs. The cargo may be selected by compatibility
and put into containers. At a specific cut off time, the full containers
can be moved together to form a cargo block. The freight ship would come
alongside, latch onto the cargo block, and begin the delivery voyage.
Initially cargo volumes would be low in accordance with demand. A young
lunar community simply would not have many people in full time residence.
Therefore a cargo vessel would need to carry perhaps five to ten 3-by-3-meter
cubes. However, considering freight voyages would occur once every week
from either terminal, a collection site would still be absolutely necessary
for customer convenience and to encourage further growth of the market.
Transit Schedule
A service based on fastest transit capable at any given time might create
weekly confusion among customers as to when to receive and prepare cargo.
Customers appreciate a reliable fixed schedule that they can plan on,
even if this means the introduction of extra contingency time for the
vessel. The goal is to create a freight service such that if, for example,
it is Wednesday, the ship must be arriving. Deciding; is it Wednesday?
Is it Apogee, what phase are we in? What time will the next ship arrive;
such ambiguous schedules do not make for customer satisfaction. Introduction
of extra time for each vessel would enable the vessel operator to handle
many unforeseen situations such that the overall schedule would not be
adversely impacted.
The customers are anyone and everyone that manages to establish themselves
on the moon: the miners, the scientists, or the commercial tourist market.
The mission of the business is to supply the transportation needs for
as wide a range of cargo users as possible. The company that takes up
this mission would be able to focus entirely on the freight system, making
it reliable, cost effective, and able to produce a sustainable profit
and growth.
Top of Page
By Terry Hancock
Traditionally, there are two major paradigms for designing space missions:
Human-crewed and Automated.
Human-crewed spacecraft use astronauts as the critical system involved
in all operations -- they leverage the tremendous versatility and intelligence
of Humans to provide a high degree of flexibility for a wide range of
mission objectives, which is why, for example, we can have a "general
purpose" Space Shuttle that we use many times over to do all kinds of
different space missions. The cost of Human spaceflight is, however, extraordinarily
high: in addition to the obvious weight of life-support equipment and
habitat modules, we must factor in the necessity of providing assured
crew return, and the essentially incalculable cost of mission reliability
to ensure crew safety. These make Human-crewing impractical for a wide
range of missions.
By contrast, automated spacecraft are quite a lot cheaper. They are
vastly smaller and lighter than crewed spacecraft, have no need of life
support or crew habitat, and can have most of their structure dedicated
to their mission. No return is required -- once they complete their mission,
they can usually be disposed of. However, they are not reconfigurable
and have rigid, inflexible mission capabilities. Furthermore, without
the intelligent and flexible Human crew to oversee them and repair them,
they need very high reliability systems: repairs during flight are typically
impossible, so they are simply designed with sufficient redundancy and
reliability of components so that they can continue to operate broken,
or have such a low chance of breaking that they can be considered safe.
This becomes increasingly difficult with longer mission durations, since
damage tends to accumulate. Since there is a high degree of interdependence,
mission software and hardware must be extensively tested together to ensure
that it all interoperates under all conceivable mission situations, and
even so, we must provide for recovery systems in the case of software
errors or hardware glitches.
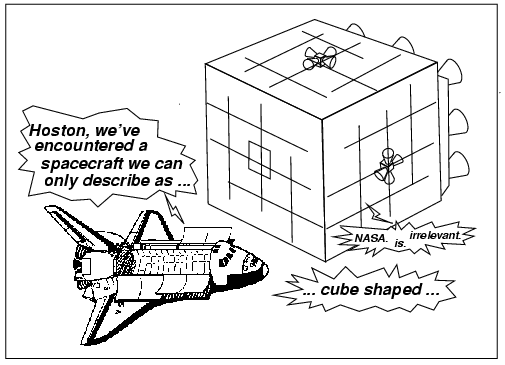 |
Cartoon by Terry Hancock. |
Since each mission is designed as an integrated whole, with tight mission
objectives and constraints, the automation must be reconceived and recreated
with each spacecraft. This results in very high spacecraft development
costs. Only the extreme mass and reliability requirements of the Human-crewed
alternative justifies this higher cost. Still, the automated spacecraft
is very specialized, and it is difficult to design for open-ended mission
concepts such as commercial freighting (where the mass and destination
are not known in advance).
What we propose is a compromise solution, which is in many ways the
best of both worlds, and in some ways better than either, at least for
a class of missions for which the other two are not well-suited. In this
solution, which we call "robot-crewing," the control complexity is concentrated
into robots specially developed for the purpose, with a modest set of
requirements on spacecraft design to accommodate robot, rather than Human,
control and repair. The spacecraft bus includes a computer and communications
system similar to that used in automated spacecraft, but generally portable
from one spacecraft to the next (a common bus design -- not a new concept).
This bus provides for support for one or more teleoperable robots, which
provide crewing functions, such as:
System gluing: independently-designed mission systems are kept separate,
with the robots acting as intermediaries, reducing the combinatorial explosion
problem associated with designing complex automated missions.
System repairs: if the systems can be designed for robot-repairability,
then the desired reliability can be achieved without having to make the
equipment itself maintenance-free.
Flexibility: general-purpose robots offer a greater range of mission
design "on-the-fly" (i.e. after the spacecraft is already in space). Since
they will have (robotic) arms, legs, and eyes, they can do many of the
things a Human crew would do to adapt to different mission concepts, instead
of requiring specialized instruments for every mission.
Better self-observation: one problem with automated spacecraft is being
able to determined the spacecraft's state, since it typically cannot look
at itself. By contrast, the robot crew will necessarily have cameras and
lighting and be reconfigurable so it is possible to image the spacecraft
itself. Thus the ground crew can have much more complete knowledge about
the spacecraft, reducing troubleshooting time.
The only downside is the increased level of communications that the
spacecraft will require: We'll need more communications bandwidth than
typical automated spacecraft. However, we can combat this by using greater
degrees of automation on the spacecraft and in the robots, and condense
the actual communication primarily to uploaded directions and downloaded
highly-compressed, selective image data. We can also simply include the
higher communications bandwidth in the overall system design as an acceptable
penalty for improved flexibility.
Reference Missions
In designing the Robot Crewing Paradigm, we have considered
several reference missions which involve relatively mild robotics challenges,
but would offer tremendous performance advantages over either conventional
automated or Human-crewed space operations, including: a translunar or
interplanetary ion freighter, an integrated cometary mining and refining
complex, and a teleoperated lunar research institute (which might include
engineering labs and an astronomical observatory).
Top of Page
Copyright © 1998-2003 Organization for the Advancement of Space Industrialization and Settlement. All Rights Reserved.
|